The academic and employer communities have developed a consensus on the broad geoscience concepts, skills, and competencies that need to be developed throughout the curriculum across multiple courses and educational experiences.
The geoscience academic community overwhelmingly agreed at the 2014 and 2016 Summits that improving competencies, skills and conceptual understanding was the most important issue for undergraduate education. Similarly, 85–87% of the 2014–2015 survey participants agreed that was very important and an additional 6–10% rated it as important. The major conclusion of the 2014 Summit that developing competencies, skills, and conceptual understanding in students was more important than taking specific undergraduate courses was also validated by the 2014–2015 survey with 81% of academics and 77% of employers agreeing. Consequently, the focus of this section is on concepts (A), skills (B), and competencies (C).
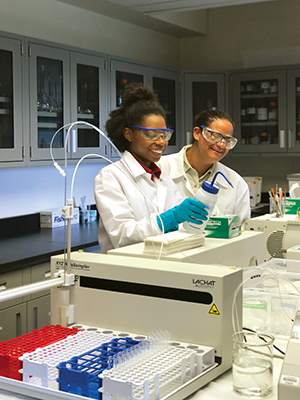
A. Core Concepts for Success
At the 2014 Summit and in the 2014–2015 survey, the academic community identified broad concepts that undergraduate geoscience majors, mainly geologists and geophysicists, should know to be successful in graduate school and the workforce. These broad concepts, ranging from core topics taught in most programs to those covered to a variable extent, are:
-
Earth as a complex system
-
Deep time
-
Surface processes
-
Earth materials
-
Earth structure
-
Hydrogeology and Water Science
-
Natural resources
-
Climate change
Both academics and employers concurred on the importance of these concepts (Fig. 3.1) (Appendix A). The 2015 Geoscience Employers Workshop participants provided even further granularity on what they thought students should know and be able to do. Many of these concepts emphasize ideas taught in traditional courses offered by most departments (such as deep time, structure, Earth materials, surface processes), while others are covered only by some programs and only to variable extents (climate, hazards, resources, hydrogeology). The employers focus was on developing an understanding of broad concepts — i.e., the processes and their impacts — to build a working framework for knowledge gained during their education and future career. Although the employers provided a robust discussion of what comprised each broad concept as outlined below, they deemphasized the importance of memorization of terms, definitions, classifications, and other material that is easily referenced online. The employers at the 2015 Geoscience Employers Workshop and participants at the 2016 Summit did not address geologic hazards as a separate concept, but instead integrated hazards into the other appropriate concepts, which is reflected in the following subsections.
1. Earth as a Complex System
Objective: Students will be able to interpret Earth’s systems, their interrelationships, and effects on each other.
Earth is a nonlinear, complex system composed of interlinked dynamic systems of different complexities of scale and interactions. These systems involve the movement of energy, solids, and fluids, commonly in cycles with different residence times. The systems and their parts interact and form feedback loops. Students need to understand the concept of systems and how they work. They must think of Earth as a system and understand its constituent interactions and forcing mechanisms. They need to consider the implications of forcings within the system and evaluate predictions. Students should particularly understand the human drivers of change and its impacts, environmental transitions, and scales over which changes occur. Students should be able to use present processes to infer past processes and use the geologic record of past processes to forecast the future, assessing the risks and advantages to changes in the Earth system.
2. Deep Time
Objective: Students will be able to comprehend the scale and magnitude of deep time, the impact of different time scales on geologic processes, and interpret its relationship to major geological and biological events in the geologic record.
Understanding geologic time is fundamental to all geosciences. Conventional means of determining relative time (superposition, cross cutting relationships, fossil succession, etc.) and absolute time (radiometric dating) should be understood, along with the precision and limitations of each of these methods. Students should be able to extrapolate from what is determined in the lab to the field and integrate this information into real geologic settings. Students need to understand the impact of time on different geologic processes. Processes can occur in real, instantaneous time, over millennia, or over millions to billions of years. The relevance of these processes depends on these different time scales. When examining Earth events, it is important that students consider rates, rates of change, duration, frequency, magnitude, residence time, scale, sequencing of events, and other impacts of time on geological processes. Examples where time is important include weathering and erosion, plate tectonics and the resultant natural hazards, the development of mineral and energy resources, and environmental changes and related hazards. Students must be able to employ temporal reasoning to address geologic problems and should recognize that different processes were more or less critical during specific periods in geologic history.
3. Surface Processes
Objective: Students will be able to recognize key surface processes and their connection to geological features and possible natural and man-made hazards.
The Earth’s surface, in both the terrestrial and marine realms, is modified by physical, chemical, and biological interactions with variable rates of physical and chemical changes. Students should understand sediment deposition and erosion processes, including transport relationships and the magnitude and frequency of surficial deposits. Subsurface analogs that cannot be measured directly should be introduced along with field exposures. Particularly important are stream and river flow and the resultant morphologies, associated deposition and erosion, and the effects of floods. Streams and rivers are the primary mechanism that shapes the continents in our current climate, a major hazard, and an important resource for humans for water, hydropower, and recreation. Familiarity with landscape alteration (or geomorphology) through mechanical and chemical processes is important, including the development of karst, glacial deposition and erosion, landslides, and wind-related surface processes. Students need to make the connections between surface processes and the Earth’s habitability and ability to sustain life. Surface processes are involved in natural hazards that affect society, and students should understand and evaluate the triggers, impacts, and risks associated with these processes.
4. Earth Materials
Objective: Students will be able to analyze and interpret the chemical and mechanical processes that are involved within each stage of the Rock Cycle.
The Earth is composed of rocks comprised of minerals with different physical and chemical properties. Students should know what rocks and minerals are, understand the rock cycle, and be familiar with the mechanical and chemical characteristics of rocks and minerals that determine how terrestrial solids behave under different conditions and change over time. Students should also know how physical and chemical properties of rocks and minerals are measured, the relevant scales of measurement, and the natural scales of heterogeneity. Knowing the processes that form rocks and minerals, the conditions of rock formation, fluid flow, dynamics and chemistry, and the role of micro-organisms on rock formation are equally important. Students should recognize the role of rock formation in the localization and development of mineral deposits and energy resources, in terms of both organic and inorganic processes.
5. Earth Structure
Objective: Students will be able to analyze and interpret plate tectonic and deformation processes, the relationship to Earth’s structure, and the resultant geological structures and natural hazards.
Plate tectonics is the central dynamic concept of the Earth system. Students need to know the different types of plate boundaries, their associated geologic and geophysical features, the driving forces of plate tectonics, and how these forces interact with other parts of the Earth system. Students must be able to link plate tectonic processes to deformation, basin formation, earthquakes, tsunamis, volcanism, and other hazards. Also important is recognizing the episodic nature and uncertainty of plate tectonic processes as they impact hazard prediction, mitigation, and planning. Central to understanding the dynamic Earth is knowing the internal layered structure of the Earth, the mechanical properties and composition of the different layers, and the various means by which we measure and define the Earth’s internal structure (seismic waves, analysis of earthquakes, analogies to meteorites, etc.). Additionally, geoscience students should understand stress and strain, rock mechanics, deformation processes, and the conditions under which different kinds of deformation occur. Students should be able to recognize the resultant structural features, including folds, faults, shear zones, fractures and joints, and know the conditions under which they form. Students should also understand the importance of structural controls on energy and mineral resource accumulation.
6. Hydrogeology and Water Science
Objective: Students will be able to analyze and interpret the chemical and mechanical processes that are involved in the Water Cycle and important aspects related to water being a natural resource.
The water cycle is a critical component of the Earth system that impacts all life and the Earth’s habitability. Students should examine the interactions among the hydrosphere, atmosphere, and land surfaces and among oceans, ice, fresh surface water and groundwater. Understanding ocean chemistry, dynamics and its layered structure is important. Students should know about groundwater and aquifers, including the difference between confined and unconfined aquifers, saturated versus unsaturated conditions, water phase behaviors and scales of aquifer heterogeneity in space and time. Additionally, students must recognize the importance of aqueous geochemistry and biogeochemical phenomena in the hydrologic cycle, including microbial interactions and nutrient cycling, contaminant transfer, groundwater quality, and impact on oceans. Discussions of economics, regulatory standards, and public policies regarding groundwater should be included.
7. Natural Resources
Objective: Students will be able to relate the distribution of natural resources to geological processes, explain how natural resources are formed, used, and extracted, and understand their relative availability.
Natural resources play a critical role for society, and it is important for students to understand the economic geology of natural resources, including water, energy, minerals, and other geologic materials. This understanding includes the economic importance of natural resources as commodities with finite availability, the economics and viability of different resources, and the distinction between resources and reserves. The geographic distribution of resources, and the geologic processes involved in their formation, extraction, and use, should be part of the geoscience curriculum. Students should have the opportunity to evaluate the pros and cons of renewable and non-renewable (finite) resources, investigate resource dependency and limits, know where materials, energy and medicines come from and how they are made, processed, or refined. Additionally, students should understand ecosystems services and their direct and indirect contributions to human well-being. Students should be familiar with the relative supply and demand for natural resources, including ore, fossil fuels, and water, and what is involved getting them to market, the scale for formation and depletion of natural resources in time and space, and whether sustainability can be achieved.
8. Climate Change
Objective: Students will be able to analyze and explain the Earth’s changing climate over various time scales and analyze the environmental, social, and geological impacts of these changes.
The Earth’s climate is changing and has throughout geologic history. Students should know what climate change is, the difference between climate and weather, how climate has changed on the geologic time scale, and how it is changing on a present-day time scale. They should be familiar with and know how to use climate proxy records (e.g., ice and sediment cores, tree rings, speleothems, etc.). Students should understand rates of change and how gradual versus rapid change creates different impacts and effects on global, continental, and local scales. Students should examine the driving forces and causal mechanisms of climate change, and how much dependence there is on the spatial and temporal scales of change. Students should also know the difference between external and internal climate forcings, what feedback mechanisms are acting, and how they work. They should consider climate change from an Earth systems perspective, including the impacts of plate tectonics, atmosphere-ocean-cryosphere-Earth interactions, the carbon cycle, the role of oceans, and human-induced climate change. Students need to analyze the impacts of climate change in the context of a variety of important societal issues: impact on water resources and the hydrologic cycle; implications for the biosphere, ocean acidification, and sea level rise; effects on soil and agriculture; impacts on ocean-atmosphere circulation; and the economics and societal aspects of climate change. Students should be aware of the climate element in environmental consulting, in hydrogeology, and in petroleum exploration.
Additionally, participants at the 2015 Geoscience Employers Workshop thought fundamental physical and chemical processes and concepts, such as thermodynamics, kinetics, diffusion, energy, heat and mass transfer, fluid flow, and key geochemical cycles (e.g., C, H2O, N, P), should be introduced where applicable to the study of the geoscience concepts discussed above.
B. Core Geoscience and Science Skills for Success
At the 2014 Summit, participants recognized that while many skills were common to science, others were specific to the geosciences. Most academics and employers in the 2014–2015 survey identified the following core science and geoscience skills as either important or very important (Figs. 3.2, 3.3).
Skills common to all sciences that students should develop include:
-
critical thinking and problem solving;
-
communicating effectively to scientists and non-scientists;
-
accessing and integrating information from different sources and to continue to learn;
-
understanding and using scientific research methods;
-
applying strong quantitative skills; and
-
working in interdisciplinary teams and across cultures.
Employers at the 2015 Geoscience Employers Workshop agreed students should be both proficient in these skills and have experience applying them. Employers felt the capability to access and integrate information from different sources while continuing to learn was something students needed to master. The 2014–2015 survey results showed that a substantial majority of both academics and employers (84–95%) viewed these science skills as important or very important, with the exception of working in interdisciplinary teams and across cultures where a smaller majority (65%) agreed (Fig. 3.2) (Appendix A).
Summit participants and employers also identified several geoscience-centric skills that students should develop (Fig. 3.3). These included:
-
making inferences about the Earth system from observations of the natural world combined with experimentation and modeling;
-
solving problems requiring spatial and temporal (i.e. 3D and 4D) interpretations;
-
working with uncertainty, non-uniqueness, incompleteness, ambiguity, and indirect observations;
-
integrating data from different disciplines and applying systems thinking;
-
applying field observation and integration skills and a working knowledge of GIS;
-
using strong computational skills and the ability to manage and analyze large datasets; and
-
being technologically versatile (i.e. Google Earth, tablets, smartphones, apps).
Of these seven geoscience skills, 80–87% of 2014–2015 survey participants saw the first four as either very important or important while 52–67% viewed the last three as very important or important (Fig. 3.3) (Appendix A). By contrast, 2015 Geoscience Employers Workshop participants felt that students should have a demonstrated mastery of five (#s 1, 2, 3, 5, 7) of these skills by having completed a project or thesis. For the other two (#4 and #6), they expected proficiency through application of these skills in their coursework or projects. Employers at the workshop stressed the importance of all these science and geoscience-specific skills, including those that garnered lower percentages in our survey responses.
C. Core Competencies
Both academics and geoscience employers agreed that developing competencies — the ability of students to use the concepts and skills they had learned — are important. At the 2015 Geoscience Employers Workshop, employers expanded on the skills identified by the academic community, identified what these skills meant in a work environment, and detailed what competencies students need to be successful in the workforce.
Employers expected, for any of the identified science and geoscience skills, at least a level of proficiency — students had experience using or applying what they knew, most likely in class exercises. For geoscience-specific skills, employers generally expected mastery — students had demonstrated the ability to use skills through a project or thesis. Only exposing students to these broad concepts with the development of some understanding was viewed as insufficient. Students must be able to use what they know.
The important competencies for skills, and to a lesser extent for concepts, that geoscience employers highlighted and were endorsed by participants at the 2016 Heads and Chairs Summit, are discussed in the following subsections. Starting with the adjacent observation from the community, and throughout this document, quotes from the heads and chairs progress reports illustrate how the results of this initiative have been applied (see also Section 12; Appendix C).
Geoscience and Systems Thinking
Employers emphasized that Geoscience Thinking and Systems Thinking are core competencies. In this context they meant that Geoscience Thinking (or geoscience habits of mind) requires thinking on geologic and real timescales, spatial thinking in 3D (and 4D), and direct field observations, coupled with geologic reasoning and synthesis (Kastens et al., 2009). When students solve geoscience problems, they need to think of Earth as a complex, open, and dynamic system of interlinked parts, processes, and feedbacks. Students need to examine how the atmosphere, hydrosphere, lithosphere, pedosphere (surface), and biosphere work as a system and interact, including the driving forces for change and their effects, and the coupled solar system-Earth interactions. Additionally, students should be able to incorporate the human element — the influence of geosciences on society and of society on Earth processes and its impact in shaping the human experience — the coupled human/societal-Earth system.
Critical Thinking and Problem Solving
Preparation for “real world” professional projects and future research requires strong critical thinking and problem-solving skills. Students need experience solving problems with authentic data and non-unique answers in the context of an open and dynamic system. Employers note that students need to understand the context of the problem and identify the appropriate questions to ask, what data to collect, and methods to use. They also need to be able to collect and compile that data, analyze its quality, interpret it, and apply their results and conclusions. What makes this process distinctive to the geosciences is the need to make predictions with limited data while recognizing and managing uncertainties. Students must have experience working on questions with no clear answers and substantial ambiguity, where they work by analogy and inference, and within the limits of certainty. To solve most geoscience problems, they also need the ability to visualize and address 3D and 4D questions involving spatial scales that may vary from atomic to global, and time scales ranging from geologic to “instantaneous.”
Employers are interested in students who have a passion for solving problems and are intellectually flexible enough to apply their skills in new situations. Conducting authentic research and the collection of new information were considered valuable student experiences. Most importantly, students needed to be prepared for and embrace life-long learning. They need to be willing to learn and apply new concepts, ideas and data, use new technology and software; and be able to critically evaluate literature so that as new information becomes available they can assess its validity and usefulness.
Quantitative and Computational Skills
Higher-level math and computer programing skills are increasingly critical for geoscientists, and competency in these skills have resulted in demonstrated increased employability and employment resiliency. Employers across the spectrum of occupations expressed the importance of these skills. Employers expect students to take calculus and statistics. The latter viewed as the most important as it relates to understanding probability and being able to analyze uncertainty and risk. All geoscience professions rely heavily on these statistical skills as most applied geoscience problems are fundamentally risk assessments.
Additionally, differential equations and linear algebra are highly recommended. Students will need to have a fundamental grasp of differential equations to effectively understand many of the key concepts in fluid flow, which is a pervasive concept in geoscience today. Linear algebra prepares students to understand how complex multivariate systems behave and how multiple variables and dependencies between them can generate multiple solutions. Employers at the 2018 Graduate Student Geoscience Employers Workshop expected that undergraduates would already have competency in all these mathematical areas. Students wishing to continue for master’s and doctoral degrees should be encouraged to start developing competency in higher-level mathematics. Another increasingly important expertise highlighted by employers at both 2015 Undergraduate and 2018 Graduate Geoscience Employers Workshops is computational methods, including computer programming and modeling.
Data Analysis Skills
The analysis and management of large datasets is increasing in importance rapidly as a competency, even more now than was evident during the 2014/2016 Undergraduate Summits and 2015 Undergraduate Geoscience Employers Workshop. In 2015, employers agreed that students need to be able to examine large datasets and statistically analyze the data to draw conclusions about the information within them; to model data using visual models, modeling tools (e.g., Stella, Modflow, Matlab, etc.), and simulations; and to integrate multiple large datasets of different types and from different disciplines. The increased emphasis on these data skills and competencies, and all levels of data analytics at the 2018 Graduate Student Geoscience Employers Workshop, was striking. Although these employers were discussing graduate students, the employers made clear that the expectations for undergraduates entering graduate school for these competencies had increased over the three-year period. Overall, employers stressed that successful geoscientists are expected to integrate technical and quantitative skills, programming, and application development. As the geosciences evolve, the need to be technologically facile and diverse, and to have strong data skills, will continue to increase.
Communication Skills
Communicating science verbally and in writing is critical. Employers at 2015 Geoscience Employers Workshops stressed that students must be able to tailor their written and verbal communication to different audiences, ranging from specialists within their field to other scientists and engineers, educated non-scientists, potential funders, management, the general public, and the press. Another key component of communication stressed by the employers is good listening skills and the ability to carry on an interactive dialogue. They noted that the best leaders know how to listen to ideas and respond constructively. Many heads and chairs in their action plan reports detailed how they embedded communication throughout their curriculum (e.g., Box 3.1).
Box 3.1: Embedding Skills Throughout Curriculum
Science communication is intentionally embedded into key parts of the curriculum, helping students increase their capacity for effective written, oral, and graphic communication to both scientific and non-specialist audiences. (Bachelor’s-granting private college)
A few examples:
-
A place-based geology project in the introductory course connects students' prior experience with their novice-level understanding of geologic processes and products. Students write throughout the semester to produce an observation-oriented geologic interpretation of a particular place that is familiar to them.
-
Evolution of the Earth (historical geology) includes both scientific writing, with a focus on outcrop- and hand sample-scale description as the basis for geologic interpretation, and public communication, in the form of museum exhibits.
-
Earth Surface Processes (geomorphology), which has always included an individual research component, now has an increased emphasis on communicating those findings to both scientific and non-scientific arguments. Students are challenged to think about real-world application alongside their scientific inquiry.
-
Research in Geology I and II, our junior-senior seminar course, in which students propose, conduct, and write a senior thesis, has an increasing emphasis on communicating to a non-specialist audience. While students continue to write a scientific paper-style thesis, they must also give a 3-minute ‘elevator’ speech to a non-specialist audience. We asked non-geology faculty to stop our students in the hallway and inquire about their work. Geology students participate in an all-campus research presentation day, in addition to the scientific research symposium that we have always done. In addition, we explicitly discuss the value of liberal arts education in shaping who they are as scientists and help them craft a personal narrative that can form the basis of a cover letter, personal statement, or interview response.
Cross-disciplinary Teamwork on Interdisciplinary Projects and Project Management
All employers participating in the workshops stressed the importance of students learning to work in teams, particularly with people from different disciplines, on projects that are commonly multidisciplinary and even transdisciplinary. In the work environment, teams comprise people with different backgrounds, specialties, experiences, and personalities. The team works together on the entire project so that this diversity of thought, experience, and specialties enhances the outcomes.
Project management in a team setting is very important. Each person must learn to be a leader and a follower, to listen and share, and to work with people who have different opinions and approaches. Teamwork requires goal setting and solution-oriented approaches, as well as understanding effective methods for addressing conflict resolution. Strategies for identifying and resolving problems as they arise are essential for projects to succeed. Students need to learn time management to assure that projects are completed in the time allotted. Effective team members need to learn to monitor their own behavior and to set aside interpersonal issues with peers, supervisors, and employees. Other important interpersonal skills are the ability to work with different personalities, emotional makeups, viewpoints, specialties, educational backgrounds, and abilities, including with people one does not like.
Field Skills
Employers strongly supported development of field skills, particularly through intensive experiences such as field camps, research projects, expeditions, and cruises. Fieldwork improves spatial cognition and creative problem solving and provides an excellent opportunity for students to gain experience synthesizing different types of geoscientific information to solve problems. Depending on the design, these experiences can also help students learn teamwork. The employers considered field experiences as unique, essential, and difficult to replicate or substitute. In some states (e.g., Pennsylvania, California) licensing for geologists requires field courses.
Essential Non-technical Skills
Many skills stressed by geoscience employers are not technical in nature but are nevertheless necessary for success. Ethical behavior and adhering to codes of conduct for professions, institutions, and employers is critical for trustworthy scientific results that guide decision-making. Also, awareness of implicit biases and the components of an inclusive environment promotes a productive workplace.
Other non-technical skills or competencies that are not specific to the geosciences but are key to success in the workforce include professionalism, demonstrating a commitment to doing an effective job, being responsible, dependable, honest, confident, committed to effective performance, time management, and generally having a professional appearance. Leadership involves effectively guiding others to accomplish goals or objectives in a coherent and cohesive manner. Business acumen, or some knowledge of the business environment that provides the ability to make good business decisions, is very beneficial. Risk management is critically important: most geoscience careers involve making decisions that include financial, environmental, structural, or other types of risks.
Geoscience is global. Not only is the science and impact global, the range of geoscience employment and colleagues are global in scale. Geoscientists must have a global perspective and the ability to work with people from different cultures. Additionally, geoscientists work on issues of importance to society, and employment in these areas is increasing rapidly. Thus, students need to develop an understanding of the societal relevance, as stressed in many of the concepts outlined above, and the ethical implications of their work.
Approaches to Developing Concepts, Skills, and Competencies
Employers at the 2015 Geoscience Employers Workshop and Heads and Chairs at the 2016 Summit discussed the best ways to develop skills, understanding of concepts, and competencies. Experiential learning was considered instrumental to achieving identified student learning outcomes, either within existing classes or as distinct experiences. Students should be constantly engaged in practicing their skills and using the concepts within classes. Gaining experience in problem solving using and analyzing real datasets is critical. Students also will benefit by using methods and geoscience equipment and tools to gather data and solve problems. Written and oral work should be integrated into classes, and intensive writing and oral presentation courses should be part of programs. Students should have opportunities to work on collaborative, integrative, and interdisciplinary team projects. Also important is interactive use of technology to gain experience with visualization, simulation, and modeling of real data.
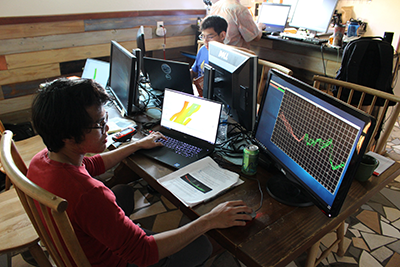
In addition to experiential learning in existing classes, substantial experiences such as fieldwork and field experiences, and capstone problem or project-oriented courses, are valuable. Independent research experiences and projects, senior theses, and internships or REUs (Research Experiences for Undergraduates) provide excellent ways to develop skills and use concepts. Employers also stressed the importance of active collaboration between academia and outside employers (discussed in Section 13).
A major concern expressed at the 2016 Summit and subsequent workshops was that most geoscience undergraduates were not prepared for the math, quantitative skills, data analysis, and computational skills recommended by the employers. At the undergraduate level, only about 25% of the graduates, and about half of advanced degree recipients, have taken substantial math beyond Calculus II (Wilson, 2018). Geoscience employers across all employment sectors, including atmospheric and ocean sciences, highlight quantitative geoscience skills as critical for bachelor’s graduates. The rapid growth of data-intensive applications in both basic and applied geoscience investigations across subdisciplines highlights the need for more advanced experiences with statistics. The ability for students to manage data and use numeric data systematically with a full understanding of the limits of accuracy and precision, and appropriate use of transformative functions and algorithms, is now central to the nature of geoscience work.
Heads and Chairs at the 2016 Summit discussed ways of developing these quantitative, data analysis, and computational skills, ranging from increasing external course requirements to integrating these skills into geoscience courses, and some cite examples of success in their progress reports (see Box 3.2; Section 12). As much of the mathematical and statistical education of undergraduates happens outside of geoscience departments and programs, it is critical that math and other quantitative skills be integrated into geoscience courses throughout the curriculum. Too often, math is not used in geoscience courses, even if it is a prerequisite or required for graduation, although the situation is improving (McFadden et al., 2019). Faculty have tried a range of strategies to ensure the quantitative abilities of their graduates, from specialized courses on mathematical applications in geology (e.g., Vacher, 2000; Ricchezza and Vacher, 2017), to developing and leveraging a range of online resources in courses to support students' quantitative skills. The NSF-supported “Math You Need, When You Need It” project (Wenner and Baer, 2015; Wenner et al., 2011) produced a set of online resources aimed at supporting student understanding of common mathematical applications encountered in introductory-level geoscience courses, such as linear regression, making and reading graphs, and understanding rates, among others.1 One specific example for both introductory and upper-level geoscience and other STEM curricula is the Spreadsheets Across the Curriculum educational resource collection2 (e.g., Vacher and Lardner, 2010) that leverage the computational functions in Microsoft Excel to help students learn different aspects of mathematics. Heads and chairs in their progress reports cited ways they were increasing the quantitative rigor of their programs (e.g., Box 3.2)
Box 3.2: Increasing Quantitative Rigor of Undergraduate Programs
Many departments have started increasing the rigor of their undergraduate programs.
One bachelor’s-granting private university came up with several different approaches to integrating more math and computational and data analytics skills:
-
All elective courses focus on developing skills and conceptual understandings valued by industry, including quantitative, written, conceptual, and technical skills.
-
We led an effort with the business and math departments to propose a new cross-disciplinary B.S. degree in GeoBusiness and Data Analytics. Students take core geology classes and courses in business, economics, statistics, big data management, spatial data analysis, programming, and technical writing. The integrated skill set developed was viewed by our advisory board and other industry experts as very strong and employable. The Academic Vice President saw it as an innovative degree that will prepare students for the business workforce and for non-geology graduate work (MBA, geo-economics, etc.). He is encouraging other departments to consider similar cross-disciplinary degrees.
-
Many of our students have an interest in geospatial studies so we created a B.S. degree that builds on that interest including core geology classes as well as and earned certificate in GIS and in another technical field including computer programming, web design, networking, or data science. Graduates with this degree have found immediate employment.
Other doctoral granting institutions have worked to overcome limited quantitative and computer skills among their undergraduates by introducing more quantitative activities across a range of courses and including examples in every course to show how these skills are important and applied in Geological Sciences. Several have started offering their own quantitative or computational geoscience courses.
Geospatial information science (GIS) skills were also highlighted as a needed addition to geoscience programs during both the 2014 Summit and 2015 Geoscience Employers Workshop. Since then, the importance of student statistical skills related to dealing with large (and geospatially controlled) Earth datasets has increased. Geospatial skills and reasoning, including spatial statistics and analysis, are highly marketable and are in high demand by students. These have been incorporated into some bachelor’s degree geoscience programs, though others recommend that students seek coursework in this area through their general electives.
Summit and workshop discussions about GIS, quantitative/computational skills, and other competencies that traditionally have been addressed through supporting science and mathematics courses centered on the lack of alignment of these offerings with the context and application needs of the geosciences. Some programs have moved to models where formal supporting courses are paired with a geoscience-focused offering in the same disciplinary area (e.g., a course in physics with a follow-on geophysics course; a course in chemistry with a follow-on geochemistry offering, etc.) to give students more context with these allied sciences.
With GIS, and in some cases quantitative/computational content, certificate programs offered at two- and four-year institutions, and/or badging, provide a mechanism for students to document these experiences and capabilities on resumes and in graduate applications. Some programs are seeking to leverage the credential trend, encountered more often in master’s programs, of stackable certificates in geoscience subfields, several of which can be compiled by students to “construct” their bachelor’s degree.
Heads and Chairs at the 2016 Summit expressed concerns that if the requirements for a geoscience degree were too rigorous, they would have problems attracting and retaining students to their majors. Feedback from the geoscience employers, however, stressed that this was doing graduates a disservice as the quantitative skills were needed for employment and the level of skills needed was increasing over time. Counter to the concern of enhanced quantitative requirements being a dissuasive factor for majors as expressed by academic participants, it is important to recognize that strong quantitative skills are a predictor of employment resilience (Keane and Wilson, 2018). Workers who completed courses in differential equations, linear algebra, and computational methods have not been displaced as quickly as individuals who lack these skills. One issue for consideration in their success, beyond the motivation for a higher level of preparation, is the cognitive development conveyed through these courses. As noted above, differential equations and linear algebra better prepare students to understand many key concepts in fluid flow and complex, multivariate systems. This cognitive development is extremely beneficial to developing a mastery of broad geologic thinking. Additionally, computational methods courses represent a core tool in appropriate data modeling and management.
Recommendations:
-
Provide students through their courses and activities the opportunity to develop an understanding of broad concepts, including processes and impacts, to build a working framework for knowledge gained during their education and future career
-
Incorporate instruction and practice in geoscience skills identified by employers and academics across multiple classes to ensure students gain sufficient competency in these skills
-
Provide students with authentic experiences that incorporate geoscience and systems thinking and problem solving (e.g., field experiences, research projects, in class exercises with real data, etc.)
-
Incorporate the development of key professional skills (communication to diverse audiences, teamwork, project management, etc.) and those skills more closely aligned with the discipline into your undergraduate program
-
Identify the key quantitative reasoning skills required for your graduates and incorporate practice in these skills at multiple points in their degree program
-
Provide students experience and practice in acquiring and analyzing real data using multiple methods and tools to solve geoscience problems (if practical for your institution) and handling large data sets, with full understanding of accuracy, limitations, and uncertainty
-
See https://serc.carleton.edu/mathyouneed for the full collection. ↩︎