Geoscience educators should further embrace active teaching strategies that research has shown to improve student learning.
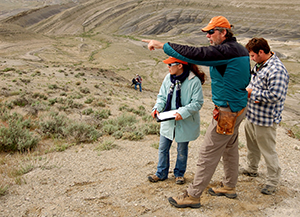
In the last few decades, new approaches to geoscience instruction have developed based on the results of discipline-based education research (Tewksbury et al., 2013; McConnell, 2019). These new teaching strategies support active learning and build on the traditional mix of classroom, lab, and field instruction to foster deep conceptual understanding of important skills and competencies (e.g., Manduca and Mogk, 2006). Many participants at the 2014 Summit were unaware of these pedagogies and the research supporting their usage. The participants concluded that the community’s primary pedagogical challenges lie in encouraging wider awareness and adoption of these practices and in characterizing their educational impacts and benefit.
Over the subsequent years, community discussions and many NSF-funded geoscience education projects, including this one, have increased awareness and adoption of research-supported pedagogies. In the Summit 2014–2015 survey, 79% of the 360 responding academics said their department was interested in making changes in how they did undergraduate teaching; 46% indicated their department was already making, or about to make, systematic efforts to encourage faculty to incorporate research-validated teaching strategies. Also, 40–53% of respondents thought that all or most of their program’s faculty were instituting some of these strategies, but that less-common strategies were used less frequently (2–22%) (Fig. 5.1). The results of the 2016 National Geoscience Faculty Survey (Egger et al., 2019) show a deeper penetration of these methods across departments with the proportion of geoscience faculty incorporating active learning instructional strategies in their courses climbing from one-third in 2004 to over half in 2016. This corresponds to increases in the frequency of use of small-group discussions and in-class exercises (Manduca et al., 2017; Egger, 2019; Egger et al., 2019; McFadden et al, 2019).
Generous NSF-DUE funding over the last twenty years has supported professional development opportunities for geoscience faculty, many through the long-running On the Cutting Edge project (MacDonald, et al., 2004), and have generated large collections of high-quality geoscience teaching resources, along with detailed “how to” explanations for a range of research-validated instructional strategies. The National Association of Geoscience Teachers (NAGT), working with the Science Education Resource Center at Carleton College (SERC; serc.carleton.edu), stewards these collections, providing geoscience instructors with free access to a rich library of shared resources that can support a transition to research-validated teaching strategies (Manduca et al., 2010; McConnell, 2019; Box 5.1).
Box 5.1: SERC and the NAGT On the Cutting Edge Program
The 17-year NSF-funded On the Cutting Edge project (2002–2019) offered a broad menu of professional development workshops for faculty[^3] and produced, aggregated, and peer-reviewed an extensive menu of teaching resources, which are available to geoscience faculty through the Teach the Earth collections at the Science Education Resource Center (SERC) at Carleton College. In 2019, On the Cutting Edge transitioned from NSF support to becoming an initiative jointly managed by the National Association of Geoscience Teachers (NAGT) and SERC. The NAGT On the Cutting Edge professional development program supports building capacity for instructional reform through the combination of professional development experiences and online materials. These programs disseminate effective curricula and pedagogical resources, provide opportunities for instructors to reflect on their teaching and learn from trusted colleagues, and promote the development of a shared vision within a community of practice (Kastens & Manduca, 2017).
The Teach the Earth portal1 provides access to online resources related to teaching and learning, and programs such as Pedagogy in Action2 are focused on describing research-based instructional strategies and explaining how they can be adapted and implemented in geoscience courses.
Major geoscience professional organizations (American Geoscience Institute (AGI), The American Geophysical Union (AGU), the Geological Society of America (GSA), and NAGT) have collaborated to create the Traveling Workshops Program3, which is intended to help guide faculty and departments through critical disciplinary and institutional changes.4
The success of these initiatives has highlighted the need for sustainable professional development experiences for geoscience instructors. To meet this need, the National Association of Geoscience Teachers established the Earth Educators' Rendezvous5 as a stand-alone, five-day professional development event, offering a mix of programming that includes multi-day workshops, mini-workshops, research presentations, round table discussions, and plenary sessions. The Rendezvous attracts more than 300 geoscience educators each summer and is an accessible forum for instructors to get a crash course in those pedagogical components that will help them to reform their coursework.
About 40% of the progress reports from participating heads and chairs at the 2016 Summit said usage of active and experiential learning increased. The most impactful action that increased usage was professional development for faculty, including attending workshops (e.g., Earth Educators Rendezvous). Some heads and chairs incorporated the use of active learning teaching into their annual evaluation process, incentivized adopting these methods, or implemented new peer teaching evaluations. Others pointed to incentivization by having enthusiastic junior faculty, peer mentoring, open faculty discussion and sharing of resources, positive outcomes supported by student assessment, and introduction of new ideas at retreats. Some recognized that changing how they taught would help student recruitment and retention.
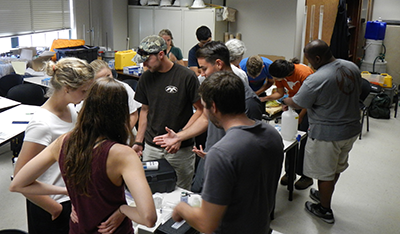
Continued progress require faculty time to implement active learning and other pedagogical innovations, such as non-instructional assignments, redistribution of workload, or release time and professional development opportunities to learn effective ways to improve student learning. Nonetheless, many of the progress reports cited resistance from faculty to changing how they taught, and many participants in subsequent workshops remain unfamiliar with most active learning pedagogies.
In the following subsections, we discuss these best practices with the goal of encouraging wider awareness and adoption of these research-validated practices and how educational impacts and benefits demonstrate the importance of integrating active and experiential learning into undergraduate programs. Faculty buy-in can be increased by using the concept of scientific teaching (Handelsmon et al., 2007), where faculty rely on evidence that shows what works in teaching.
Active Learning and Building Content Knowledge
Active learning in the classroom builds a deeper understanding of geoscience concepts. Students learn better when they can actively monitor their understanding through a variety of activities during class (Pollock & Finkelstein, 2008; Derting & Ebert-May, 2010; Freeman et al., 2011, 2014). Adopting these empirically validated instructional practices also increases student retention rates (Russell et al., 2007; Graham et al., 2013) and reduced achievement gaps among different student populations (Haak et al., 2011; Eddy & Hogan, 2014).
Active learning environments typically include two or more of the following elements:
-
Students observing or participating in activities in addition to, or instead of, listening to direct instruction;
-
Opportunities for student reflection on their learning, or student/instructor interactions that lead to assessment of learning;
-
Peer-to-peer interaction among students as they complete activities (McConnell et al., 2017).
Supplementing or replacing lectures with in-class exercises and small group or entire class discussions allows students to think about and use lecture information and to learn collaboratively. In the geosciences, courses with a high level of active learning typically include exercises with the interpretation of maps, graphs, and/or natural datasets (Teasdale et al., 2017).
Many research-validated methods for engaging students in active learning are widely used in both large and small-enrollment courses. McConnell et al. (2017) discuss the instructional utility and learning efficacy of several of these strategies (discussed below) and summarizes the supporting research. Many of these methods have resulted in improved conceptual learning and retention of specific knowledge, and most have high utility — they are relatively straightforward to prepare and use in almost any class setting. Many of these same methods have had positive impacts on learning, supported by multiple studies across several settings. Instructors who revise a geoscience course may prefer to start by incorporating some of these higher utility strategies as there are many readily available geoscience-specific examples.
The three easiest to use strategies are Think–Pair–Share, minute papers (Box 5.2), and peer instruction (Box 5.3), all of which involve students responding to questions in class and are useful in classes of any size. Think-Pair-Share is commonly used to generate class participation and social interaction between students and can be used as retrieval practice. Minute papers provide a good way to check and correct understanding during lecture.
Box 5.2: Easy to use Active Learning Practices.
Think-Pair-Share6. Students are presented with a question and asked to think about their answer, discuss it with a neighbor, and then engage in a full-class discussion. This exercise is particularly useful in large classes and increases constructive social interaction among students. Students may feel more comfortable asking questions and participating in class discussions once they know others have the same questions or understanding of the material. Depending on what is asked, it can also serve as a retrieval practice, where students are asked to recall and use something they learned previously. Although Think-Pair-Share is easy to use, most research studies that have included Think-Pair-Share have done so in combination with other active learning strategies, and there are limited research on the effectiveness of this strategy used alone.
Minute papers7. Students are asked to briefly write about what they thought was the most important thing covered during the lecture or what was the most confusing. To be effective, the instructor needs to use the results in the next class period to confirm what was important or clarify what students found confusing. Minutes papers are generally straightforward to implement and are moderately effective at improving learning.
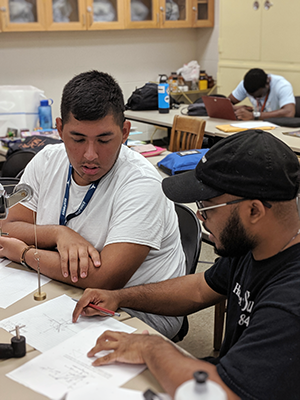
Peer instruction strategies are one of the most widely adopted active-learning approaches (Crouch and Mazur, 2001), and are well suited to assess students' comprehension of concepts, or their application of concepts to new situations. In the geosciences, ConcepTests (McConnell et al., 2006) are available through SERC and can be readily incorporated into most class settings. Lecture Tutorials are also available but involve more upfront preparation (see Box 5.3). These approaches allow students to assess their learning, identify the concepts and content that are important for the course, and develop communication and reasoning skills among their peers. These strategies help instructors quickly identify the content that most students understand or topics that present a challenge and allow instructors to adjust in-class activities accordingly. Peer instruction has high-to-moderate utility, depending on the specific approach, and its effectiveness in improving student learning is well documented8.
Box 5.3: Peer Instruction and Lecture Tutorials
Peer instruction. These activities are built around lectures and divided into segments, punctuated by multiple-choice questions (ConcepTests) that focus on key concepts. The students “vote” for the correct answers individually, via clickers, a learning response system such as Learning Catalytics, or a show of hands. If a high percentage choose the correct answer, the class moves on. However, if the question draws a mixed response (i.e., correct responses between 35% and 70%), students are directed to discuss the reasoning behind their choices with neighbors or assigned group members, and then they vote again based on the results of that conversation (Mazur, 1997). Typically, the instructor then discusses the explanation for the correct or best answer before moving on to the next topic.
Lecture Tutorials. The instructor delivers a short lecture on a concept, followed by students working together on a set of relevant questions with the instructor and teaching assistants circulating through the class to provide guidance and support to the individual groups. Group work is followed by an instructor debrief explaining the best answers and answering questions.9
Other active learning strategies often involve more effort and preparation but are recognized as very effective at improving learning. These include teaching with models, constructing concept maps, case studies, and problem-based learning activities. The common practice of physical models being used during lecture demonstrations, and/or by students in lecture or lab settings, has been shown by research to engage students and improve learning. Concept maps, where students graphically represent what they know about a topic and show similarities among various ideas and concepts, also has been shown to improve learning10.
Case studies11 and problem-based learning exercises12 have the potential to examine real-world science and emphasize application and problem-solving rather than memorization. Such activities permit instructors to engage students in higher-order learning and to address problems that involve the analysis and/or synthesis of large datasets. Using real data, where students work on problems with no exact answers, either in class or in lab, is important for developing problem-solving skills and increasing conceptual understanding. Such exercises may involve case studies that model a specific situation, requiring an action in which students take on different roles reflecting a real scenario (e.g., representing a company, a member of the community, a geoscientist, etc.), encouraging the development of communication and non-technical skills as well as content-specific understanding. These activities commonly involve students working together in small groups on questions while the instructor and/or teaching assistants provide guidance. Again, the activities are followed by a debrief as the instructor solicits ideas from student teams before revealing correct or (in the case of real-world scenarios) historically accurate explanations as to how the issues were addressed and answering student questions.
Case-based or problem-based resources are rarely presented in textbooks, but a growing collection of online resources, hosted at SERC, emphasize the relationship between the geosciences and society, and provide opportunities for instructors to use activities that incorporate real world data13. Additionally, some employers are willing to provide datasets and real-world problems for classroom use.
Active Learning in Developing Skills and Competencies
Active learning strategies also develop key skills and competencies, but unlike geoscience content that may be specific to an individual course, skills need to be revisited in multiple classes so students can practice, develop competency, establish mastery, and recognize how these proficiencies are employed. Geoscience instructors should work toward having learning outcomes that target broadly applicable skills and competencies in data analysis, scientific communication, quantitative reasoning, and geoscience and systems thinking.
Scientific communication skills develop only through repeated practice with different types of professional written and oral presentations. Scientific abstracts, “one-pagers” for non-geoscientists, and short grant proposals provide students with a variety of written formats targeting different audiences, while keeping the evaluation and review process manageable for the instructor. Multiple short assignments involving review and editing in several courses provide more useful communication practice to students than term papers. Similarly, short student presentations in classes targeted to different audiences provide students with chances to improve their presentation skills. Although technical writing classes can be effective, it is important to integrate these skills throughout the geoscience curriculum.
Geoscience thinking involves spatial thinking, particularly visualizing in three dimensions from field observations, temporal reasoning, and understanding Earth as a complex system (Kastens et al., 2009). In both introductory and majors' courses, students need practice solving 3D spatial and geologic time problems. Students need to be able to describe a natural system in detail, discuss changes that have multiple effects, and recognize and analyze feedback loops. All geoscience students need experience making field observations, conducting field-based investigations, and working with geospatial data.
In the 2016 National Geoscience Faculty Survey, geoscience faculty reported that approximately two-thirds of their students work with geospatial data at least once during their courses, and more than half indicated that students made field observations (Egger, 2019). Similarly, most instructors, whether teaching introductory or majors' courses, reported that their students practiced both 3D spatial thinking and temporal thinking at least once, with this kind of practice occurring more commonly in majors' courses. Likewise, a majority of instructors indicated that they featured some aspects of system thinking (e.g., describing a system, discussing a change with multiple impacts) in their courses, while other features of systems (e.g., analyzing feedback loops) were typically not covered.
Although a large proportion of geoscience instructors self-identify as teaching with a focus on a range of skills and competencies (Viskupic et al., 2020), the evidence is less clear as to whether the necessary learning outcomes are successfully achieved. Assessing content knowledge is easier than measuring the development of specific skills and competencies which necessarily develop over multiple courses throughout a curriculum across several years. Community college (2YC) faculty can play an important leadership role in developing best practices for geoscience education. 2YC faculty have a greater capacity to trial-run and enhance active learning pedagogies given the number of opportunities a 2YC professor has to teach introductory courses in one semester (typically 5–7 per semester). 2YC institutions have more diverse student populations, relative to most geoscience programs at four year institutions (4YC), and as such can better represent a broader perspective of the experiences of minority students. Finally, the 2YC faculty focus predominately on teaching and learning, and less on research and publications, allowing more time to develop effective pedagogy. As we continue to rise to the challenge of improving geoscience recruitment, education, and retention, collaboration between institution types and their faculty are a key component to our success.
Laboratory Learning Settings
The laboratory environment provides excellent opportunities for students to address problems or questions that require them to think and act like scientists. Lab experiences should help our students develop a mix of technical skills (e.g., map reading, mineral identification) and scientific reasoning skills (e.g., interpreting the geologic history of an area from a cross section). Lab activities can vary from relatively basic exercises in introductory courses (e.g., using geologic and topographic maps to answer questions), to more sophisticated tasks in majors' courses such as research, experimentation, fieldwork, or modeling and simulations. Conducting inquiry-based activities in laboratory courses can promote student-centered teaching that emphasizes the process of scientific inquiry and focuses on the student’s role in investigating scientific questions and building conceptual understanding. Inquiry-based lab activities in geoscience courses improve student learning in comparison to more traditional lab exercises (Grissom et al., 2015; Moss and Cervato, 2016). Based on the 2014 –2015 Summit survey, most active learning occurs in course-related labs, with 53% of faculty saying most to all labs in their program are inquiry based (Fig. 5.1).
Inquiry should engage in scientifically-oriented questions, give priority to evidence, formulate explanations from evidence, connect explanations to scientific knowledge, and communicate and justify explanations (NRC, 2000). Students are unlikely to experience fully authentic inquiry (formulate their own research question, design their procedures, analyze and communicate their results, and formulate conclusions) except in selected upper level courses or in specially designed research-experiences. Instead, lab activities should run the from low-level inquiry such as basic confirmation tasks (e.g., students identify a mineral or read a map elevation) to higher level open inquiry tasks that provide students with a question and some background information and expect them to design their experimental procedures and decide how to analyze and communicate their results (Ryker and McConnell, 2017).
The level of inquiry should match the task at hand. A review of activities in four published lab manuals for introductory physical geology courses revealed that most (~88%) of the included activities involved relatively low levels of inquiry. Limiting students to low-inquiry activities may influence their views of the nature of science as a primarily confirmatory, fact-gathering activity, and may negatively influence their perceptions of geoscience. Lower-level inquiry activities are suitable for descriptive tasks while activities with higher levels of inquiry are more appropriate for more complex and abstract concepts. By providing a mix of high- and low-inquiry activities in introductory geology laboratory courses, students develop a better understanding of geoscience and of the nature of science. Students who have positive experiences in introductory level science are more likely to persist and take a second course in the discipline. Although tasking students with completing higher-level inquiry activities may cause frustration, scaffolding the learning for those students so they can engage in these activities can lead to a sense of accomplishment, improved theoretical understanding, and a view of science as a creative process by which we investigate the world around us (Ryker and McConnell, 2017).
Experiential Learning
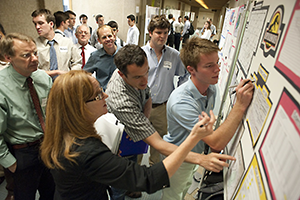
Experiential learning is an effective way that students gain skills, knowledge, and experience outside of the traditional academic classroom or associated laboratory setting. These experiences take the form of co-curricular activities such as internships, studies abroad, Research Experiences for Undergraduates (REU) and service-learning projects. Field trips and courses, field research, senior or honors theses and independent research projects also provide first-hand experience for students to learn concepts and develop proficiency or mastery of geoscience and science skills. Most experiential learning activities offer opportunities for authentic inquiry. Conducting research develops critical thinking and problem-solving skills while developing a fuller understanding of content knowledge. Field courses and field camps commonly include multiple types of group projects that, in addition to making direct field observations and building team skills, require thinking on geologic and real timescales, spatial thinking in 3D (and 4D), and geologic reasoning and synthesis. Many of these courses also include written or oral presentations.
Another approach to experiential learning is course-based research experiences (NASEM, 2015). Some of these build entire classes around collaborative, interdisciplinary team-based projects, providing experience in project management and teamwork. Such courses are often capstone experiences, like engineering design courses. These courses can incorporate service learning, research projects, or collaboration with local geoscience employers (sometimes associated with internships). Other examples include courses designed around field-based investigative projects (see Box 5.4) or modeled after the AAPG Imperial Barrel competition14 where student teams do a prospective basin evaluation, analyze a dataset (geology, geophysics, land, production infrastructure, and other relevant materials) and prepare a 25-minute presentation on their results. Some departments have developed a different approach where a large student project (individual or team based) spans multiple courses, and students work on different aspects in each course. In the 2014–2015 survey, 30% of respondents reported that one or more project-oriented courses were offered in their departments (Fig. 5.1).
Box 5.4: Marine Geology and Geophysics Field Course: Experiential Learning Through Collaborative, Interdisciplinary Team-based Field Project
Each Maymester, the University of Texas Institute for Geophysics (UTIG) offers a marine geology and geophysical field course designed to provide hands-on instruction for upper-level undergraduate and graduate students in the collection, processing, analysis, and interpretation of marine geological and geophysical data. The class is taught by three researchers with three marine technicians who act as the teaching assistants and oversee safety training and instruction.
Initially, the class provides students with three days of classroom instruction on the theory for the different techniques. The class then travels to the Gulf Coast for a week of at-sea field work and on-shore lab work. Students gather multibeam bathymetry, sidescan sonar, high-resolution seismic reflection and chirp sub-bottom profiling data, and take sediment cores and grab samples. In the lab portion, they analyze their geophysical data and the sedimentology of resulting seabed samples (e.g., core description, grain size analysis, x-radiography, etc.).
Upon returning to Austin, students work in teams to integrate data and techniques into a final project that examines the geologic history and/or sedimentary processes as typified by a small area of the Gulf Coast continental shelf. Students spend one week learning interpretation methods using industry-standard, state-of-the-art software (Focus, Landmark, Caris, Fledermaus). On the last day, students present their final project to the instructors, their fellow classmates, and foundation and industry sponsors.
Alternative Learning Settings
Another type of instructional reform focuses on altering the course structure itself by introducing opportunities for learning outside the confines of a typical classroom through the addition of online components. Such changes are facilitated by the prevalence of online learning management systems (e.g., Blackboard, Moodle, Canvas) and the availability of extensive suites of free resources to support geoscience instruction15, especially in introductory courses. One challenge for instructors seeking to use active learning strategies in their classes is finding the course time to incorporate these strategies without sacrificing content coverage (Crouch & Mazur, 2001). This dilemma has contributed to the development of the “inverted” or “flipped” learning model that places some of the responsibility for learning basic content on students outside of class time, which is often facilitated by the use of quizzes and on-line assessments (e.g., Lage et al., 2000; Bishop and Verleger, 2013; Gross et al., 2015). This ensures students have a stake in interacting with the basic content, and, by moving some content outside of the classroom experience, provides additional class time for students to tackle more demanding activities with the instructor and peers (Strayer, 2012; Gajjar, 2013). Additionally, the practice of “flipping” may allow the instructor to explore topics in greater depth, and present students with opportunities for formative assessments so they can practice applying a new concept and assess their comprehension (Freeman et al., 2011). Implementing such classroom approaches involves substantial initial logistical planning.
Box 5.5: Examples of "Flipped" Classes
2-year Community College:
I have successfully transitioned to a flipped classroom model, which focuses on active learning vs. passive listening. I use only open educational resources (OER) in my introductory courses and have developed exercises that fully utilize classroom time to explore topics through investigation using a plethora of physical samples and models plus technology using large data sets, interactivity, animations, and video instruction. I assure that students leave my introductory courses with the skills necessary to analyze geoscience related data to assist them in their future course of study and in their everyday lives.
Doctoral-granting R1 Public University:
Peer mentoring works in active-learning classrooms. “New” faculty co-teach these “flipped” courses with seasoned instructors and then take over as lead instructor after 1–2 semesters. This has been successful with three faculty in our introductory course. With the help of a teaching postdoc, we also “flipped” the associated lab course. Metrics for this introductory course show the transformed format promotes success for underrepresented minorities (URM) and women, and URMs in our major have increased over the time-period of the transformation. We implemented new peer teaching evaluation that includes teaching practices inventory.
Initially, this process was done for an introductory course, however, they are slowly drawing more faculty into transformed courses and these experiences appear to “trickle” up to their major courses. Mineralogy, Geochemistry, and Biogeochemistry are now also taught as “flipped” class. The mentoring approach used within the transformed courses was very successful. Student evaluations for these instructors were immediately high without any of the dips seen with other implementation strategies. A new graduate training course, with specific training in active learning, greatly improved TA support in these types of courses and will be expanded this year to include more strategies for student success (time management, etc.).
Use of Technology in Teaching and Learning
Technology is increasingly used for instruction and to transform the structure of classrooms (Whitmeyer et al., 2016). Creating 3D visualizations and/or animations, either as models of geologic features or from real data (e.g., maps and cross sections), which can be rotated or viewed as 2D sections in any orientation, greatly increases students' ability to visualize and understand complex geologic structures, features, and spatial relationships. Witnessing major geologic events virtually through time-lapsed videos enhances student understanding of geologic processes. The development of virtual field trips and lab experiences makes it possible for many students to have experiences their institutions cannot provide. Thus, internet and information technologies have evolved as instructional tools from “eye candy” into sophisticated systems that facilitate student exploration and classroom interactivity. The major advances taking place in visualization and geospatial tools, generation and use of massive amounts of quantitative information, and computational modeling and simulation provide both predictive capabilities and insight into processes and global-scale events.
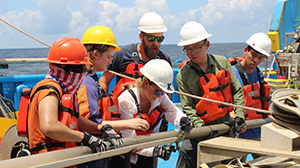
In the 2014–2015 survey, most respondents (94%) indicated that faculty used passive observation of visualizations in the classroom, however, many (>60%) were using technology that actively engaged students in classroom settings. Virtual fieldtrips, student driven investigation with real time feedback, and social networking, games and crowdsourcing had under 31% penetration (Fig. 5.2). With the onset of the COVID‑19 pandemic and the canceling of many 2020 field camps and courses, the community has greatly accelerated the development of online field exercises, simulations, and computer “games”16.
In standard field courses, many institutions already incorporate technology. In the 2014–2015 survey, up to 60% of faculty respondents said technology was used in conjunction with fieldwork, ranging from mapping on tablets or phones (23%) to using ArcGIS or other similar software (49%), to the instructor (60%) or student (35%) preparing or developing information in advance from remote sensing, Google Earth, DEMs, etc., prior to conducting fieldwork (Fig. 5.3).
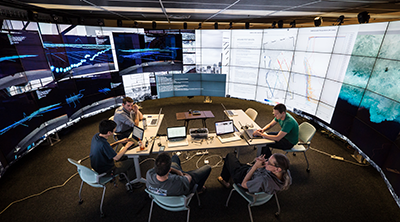
The 2014 and 2016 Summit participants discussed the evolution, potential, and challenges of technology in teaching and learning, and little had changed between the two meetings in terms of usage. With some notable exceptions, the geoscience community has not fully embraced the potential of such approaches, including social networking, educational games, and crowdsourcing, to effectively engage with a generation of students immersed in digital information and online social interactions. Community-wide strategies for developing and integrating appropriate technological advances into future educational efforts (e.g., leveraging the shift to mobile devices, harnessing data for educational research, or developing virtual communities of practice) are needed. One positive outcome of the COVID‑19 pandemic has been a significant increase in such development and sharing of such resources between educational institutions with local customization.
Recommendations:
-
Instructors should become conversant with, and adopt, active learning teaching strategies that research has shown to motivate students and enable improved learning.
-
Incorporate activities that develop skills and competencies in multiple classes so students can practice, establish mastery, and recognize how these proficiencies are broadly employed
-
Conduct inquiry-based activities in laboratory courses to emphasize the process of scientific inquiry and focus on the students' role in investigating scientific questions and building conceptual understanding
-
Use current and emerging technology and computational models and simulations using large datasets to increase student understanding of complex geologic structures, features, and spatial relationships and to provide insight into processes and global-scale events
-
Request or provide, depending on your role, opportunities for faculty to implement active learning and other pedagogical innovation — such as time, redistribution of workload, non-instructional assignments, and professional development, according to individual institutional policies
-
See full listing of the NAGT On the Cutting Edge services and support at https://nagt.org/nagt/profdev/oce.html ↩︎
-
https://serc.carleton.edu/introgeo/interactive/tpshare.html ↩︎
-
https://serc.carleton.edu/NAGTWorkshops/teaching_methods/conceptests/ ↩︎
-
https://serc.carleton.edu/sp/library/lecture_tutorials/index.html ↩︎
-
For Concept Maps: https://serc.carleton.edu/NAGTWorkshops/assess/conceptmaps.html; also see McConnell et al., 2017. ↩︎
-
For case studies: https://serc.carleton.edu/sp/library/cases/index.html; https://serc.carleton.edu/NAGTWorkshops/undergraduate_research/case_studies.html ↩︎
-
For problem based learning: https://serc.carleton.edu/NAGTWorkshops/problem_solve/index.html ↩︎
-
For example, InTeGrate https://serc.carleton.edu/integrate/; GETSI, https://serc.carleton.edu/getsi/. ↩︎
-
For example, the Teach the Earth portal at SERC: https://serc.carleton.edu/teachearth ↩︎
-
https://serc.carleton.edu/NAGTWorkshops/online_field/activities.html ↩︎